|
|
How Do Vaccines Work?
By Simon Menanteau-Ledouble
Posted on: 8/14/2011
|
This article was originally published in four parts at SheThought.com, and is republished here thanks to the author.
I. Acquired humoral immunity, part 1: the origin of immunoglobulin Vaccines work by taking advantage of the natural mechanisms for acquired humoral immunity, a really cool mechanism in itself. To summarize: our genome contains three loci of genes (two of these loci code for what is called the light chain of the immunoglobulin while the third one codes for the heavy chain).
The heavy chain is composed of three main regions of interest: the V, the D and the J region (V stands for ‘variable,’ D for ‘diverse’ and J for ‘joining’). Interestingly, each region contains multiple, slightly different copies of the same segment (a bit like the AC/DC discography). For example, we have a bunch of V segments following each other, and then a bunch of D segments and finally a handful of J segments (in total, we have 65 copies of the V segment, 27 of the D and 6 of the J segment stacked next to each other). The light chains are pretty similarly organized but lack the D segment.
Now, there is a group of immune cells referred to as lymphocytes. During their maturation process they undergo what is called somatic recombination. Simply put, it consists of the random elimination of all but one segment of each kind of region. This generates a random sequence of this segments. In addition, random nucleotides are added in the process at the VDJ joint, a process that increases the diversity even further.
Thankfully, Wikipedia provided us with a nice little diagram of the process:
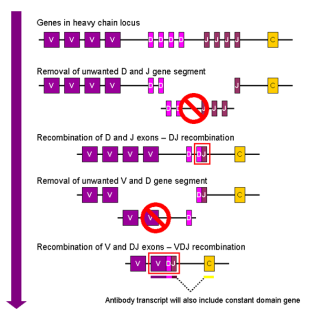
Figure 1: Somatic recombination (Most images can be clicked for larger size.)
The immunoglobulin molecule is then assembled using two light and two heavy chains, assembled together by disulfide bonds (figure 2) in such a way that the highly variable region created by the somatic recombination in each chain stick together in two regions called, quite unsurprisingly, the variable region.
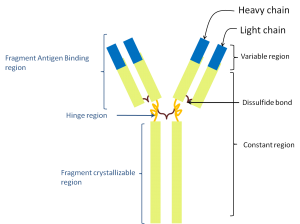
Figure 2: The structure of the immunoglobulin molecule.
This process is a veritable machine to create diversity. The recombination of random versions of the multiple segments, itself, will produce about three million different possible combinations. Introduce the additional layer of random nucleotide insertion and the number of possible variable regions jump to an astonishing estimated 1016.
Now, this change occurs within the cell’s genome. So each cell will only produce one type of antibody with its own particular sequence. But taken together, this amazing diversity ensures that, whatever molecules are expressed by the pathogen entering the body at any given time, there will be a lymphocyte expressing just the right antibody to match it.
II. Acquired humoral immunity, part 2: the various types of lymphocytes In the last chapter, we talked about the lymphocytes collectively. However, as usual, nature is more complex than that.
Essentially, we have two big groups of lymphocytes, defined chiefly by where they finished their maturation. The first one is composed of the lymphocytes B, or B-cells, so called because they mature in the bone marrow (or in birds, the bursa de fabricius. You too can become a hoot at parties by offering to show your bursa de fabricius to random strangers). The second group is called T-cells because, they finish their maturation in the thymus.
Finally, there is a third group of lymphocytes, composed of natural killer (NK) cells. These do not undergo the somatic recombination and do not produce the antibody-like receptor we mentioned previously. Instead, they check the surface of passing cells for the presence of markers of self, including MHCI. If such markers are absent, it is a sign that something very wrong is going on inside. Either the cell is infected by a virus or it is cancerous. The NK cell will therefore either signal the cell to enter apoptosis or simply secrete molecules that will attack its cell membrane and lyse it. There is some evidence that these cells also have some kind of long-term response but this is new data and a bit messy, so we won’t be mentioning it any further.
So, we have the B-cells and the T-cells that themselves include two groups, the T-helper cells and the cytolytic T-cells.
The B-cells, once activated, specialize into plasma cells, veritable little factories specialized in producing antibodies. The T-helper cells are so called because they secrete a bunch of signals that help coordinating the immune response. Of special interest for us is their role in activating the plasma cells.
The cytolytic T-cells are pretty similar to natural killer cells in function except that they do not check for the absence of the MHC markers. You see, markers of the MHC routinely sample products synthesized inside the cell, therefore, by checking the products expressed on the MHC the cytoloytic T-cell is able to glimpse what type of molecules are being produced inside. In particular, it will show if viral proteins are being assembled, signaling to the cytolytic T-cell that it is time to strike (for truth, justice and the immune system).
III. Acquired humoral immunity, part 3: enter the antigen As we mentioned at the end of the previous section, each pathogen, and indeed, a number of non-pathogenic organic substances, will express molecules that match the various immunoglobulins produced. The molecules that pathogens express and the immune system ‘recognizes’ are called antigens and, because pathogens express more than one type of antigen, the various antigens expressed, taken together, are referred to as the antigenic profile (figure 3).
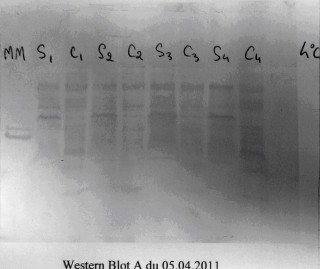
Figure 3: This Western-blot prepared by a student of mine displays the various antigens present in a bacterial cell wall.
At this stage, the immunoglobulins produced stay stuck on the surface of the cell that produced them. That way the leukocyte ‘recognizes’ the antigen it corresponds to. However, because these molecules are so specific, the odds of a circulating leukocyte encountering its corresponding antigen are very low (it is estimated that, for one specific antigen, less than one in 10,000 or one in 100,000 lymphocytes will recognize it).
However, these lymphocytes also re-circulate between secondary lymphoid organs (spleen, lymph nodes and discrete locations around the gut and respiratory track called GALT and BALT, respectively). When a cell of the non-specific immune system (which is not as picky and is quite likely to identify any given antigen) capture one such antigen, it migrates to the secondary lymphoid organ to present it to the lymphocytes. Because, as mentioned, lymphocytes congregate in these organs, the odds of finding one matching any given antigen are drastically increased.
It’s a bit like forgetting something on the bus. Your odds of finding it back riding the bus system are pretty low, but if you hang out at the lost-and-found, there is a reasonable chance that somebody will bring it there for you (I guess that comparison means that we will all be in deep trouble when leukocytes start learning to pawn antigens for booze money).
In the germinal centers, the dual presence of an antigen B-cell’s receptor and of signaling from activated T-cells will activate naïve B-cells (a naïve cell is one that has not been activated by an encounter with an antigen) that will undergo somatic hypermutation, essentially, one more step of point mutations. These mutations will be slight changes in the sequence of the variable region. As you’d expect these changes can either bring the sequences closer or further away from a match with the antigen. So this hypermutation can either increase or decrease the strength of the binding to the presented antigen but the cells in which the strength of the binding is decreased enter programmed cell death. So the outcome this process allows for the production of cells with yet even more specificity to the antigen. These cells will then switch to a different class of antibody, called IgG (see figure 4). This new class of immunoglobulin is both smaller and more specific and constitute the main thrust of the specific immune response; the one that really gets out there and kick asses.
Another thing that takes place in the germinal center is a clonal expansion of the plasma cells. There the cells that, as we have said, had already been selected to be highly reactive to the infection taking place, greatly multiply in number. Among the cells produced at this time are memory B-cell that will then move into the bone marrow when they where they will survive for several years (in comparison; estimates for the duration of circulating plasma cells range from only a few days to a few weeks).
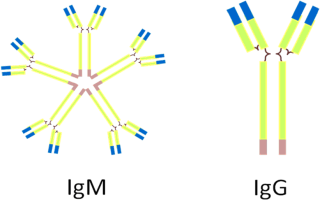
Figure 4: The two main types of antibodies.
IV. Acquired humoral immunity, part 4: the memory cells and vaccines As we have seen, mounting the adaptive immune is a complex process and it is also quite slow. Generally, it takes between ten days and a few weeks (a couple of weeks on average) for the body to mount an effective specific immune response.
But after this, the body will have a stock of highly specific memory cells remaining so that, if the same antigen is encountered a second time, the immune response will be able to bypass these steps and will start chucking out antibodies much faster.
This is particularly important as the first days of the infection are the most critical: the pathogen’s numbers are at their lowest but, because the body immune’s response is as its weakest; they are also increasing at their fastest rate. By cutting the response time, the body is therefore able to counter the pathogen before it was able to establish an infectious beachhead.
Now, you’ll notice that, when describing the mounting of the immune response in part two, I used the term antigen but never mentioned the actual pathogens it is normally attached to. That’s not an accident, because any molecules that trigger the immune system will be processed through this process. Whereas an actual pathogen or not, active or not, virulent or not, the basic mechanism behind the immune response will be the same.
So, that’s how vaccines work. Getting the non-specific immune system to pick up some antigens and get some good ol’ fashioned clonal expansion going on, knowing that out of the cells produced then, some will be memory cells.
V. Acquired humoral immunity, part 5: compartmentization of the immune system So, we mentioned how the B lymphocytes made a circuit through various secondary lymphoid organs where they could be activated by exposition to antigens. This is important because it is the cause of compartmentalization of the immune response.
Basically, one can define three major compartments of the body and match them to particular secondary lymphoid organs. For example, the lymph nodes are present located in small patches in the groin, armpits and neck (you know when you go to your physician for a fever and he palpates under your jaw? He is checking lymph nodes there). The spleen collects antigens brought there by the blood stream and all along the digestive and respiratory track you can find a bunch of Mucosa Associated Lymphoid Organs.
Now, naïve lymphocytes are released in the blood and, from there, circulate between the various secondary lymphoid organs. If the lymphocytes fail to get activated there, they will finish their routes and return to the blood. However, if they get activated in a secondary organ, the surrounding signal will control the secretion of matching receptors called lymphocyte homing receptors. For example, if a lymphocyte is activated in the spleen, it will secrete receptors that will help it bind cells of the spleen. If it is activated in a mucosal associated tissue, it will bind to other cells of the MALT. This phenomenon is called the homing of the lymphocyte and allows for the lymphocyte to remain in the compartment of the immune system it was activated in.
Such specialization makes sense, having your immune system trying to get rid of your gut flora, for example, is a recipe for disaster; the bacteria there are an integral part of your digestion process. On the other hand, if you ever end up with E. coli in your bloodstream, you will want your immune system to get off its leukocytic butt and get rid of them as fast as possible.
The various compartments are not perfectly segregated, there is a bit of communication between them, especially from the lymph nodes and GALT toward the blood stream but this communication is limited.
Finally, while we are on the subject of the mucosal immunity, it is a good opportunity to mention yet another class of antibodies, in addition to IgM and IgG: the IgA. While IgM was pentameric and the IgG monomeric, the IgA is dimeric (see figure 5) and is, if you want, composed of two immunoglobulin molecules linked together by a short polypeptide chain called the J chain (for joining chain).
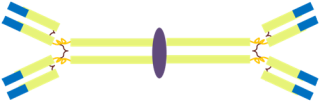
Figure 5: The structure of the IgA molecule.
VI. Making vaccines And, we have seen, the adaptive humoral immune system works through the clonal expansion of cells that match the antigens encountered. Furthermore, this expansion also produces a small number of long lived memory cells that allow to shorten the response time upon re-exposure to this antigen. Vaccines, therefore, are simply a way to arrive to this step without the uncertainty and disagreement of the infection that normally brings the antigens to the immune system by supplying these antigens through a safe and controlled method. There are a few different ways of doing that
Use of closely related agents:
As we mentioned, by providing antigens from one disease agent to the immune system, one can select the immune cells that will react against this antigen.
But, it is important to note that such antigens are not necessarily unique to one species. Closely related species can express antigens that are similar, or at least similar enough that the corresponding T and B cell receptors will work equally for both. Therefore, by vaccinating with an agent, one can confer protection against another, similar, agent. The trick, of course, being to use a less virulent agent to vaccinate against a more lethal one.
This is how vaccines were first discovered, when Edward Jenner, after noticing that milkmaids — often infected with cowpox — were resistant to smallpox, was the first to report the protective effect of exposure to Vaccinia virus against smallpox infection. It is now known that the vaccinia virus, the cowpox virus and Variola virus (the causative agent of smallpox) all belong to the same Orthopoxvirus family. These viruses are simply close enough that the cells raised by one would still recognize the others. (As a side note, it is often said that Jenner used cowpox virus, in fact, when we looked at it more closely, decades later, it was discovered that Vaccinia was a distinct species. It is however hypothesized that the strain may have initially indeed been a cowpox virus and that it just mutated since Jenner’s 1796 report.)
From a practical point of view, the use of such method of vaccination is, however, very limited. First of all, nature needs to provide you with a non-virulent but conveniently closely related organism. Moreover, the organism in question is still a pathogen in its own right, if not a proper human pathogen, as such it can cause some side-effects, especially in immunocompromised patients. Vaccinia for example, can cause some rashes or fever and even, in rare cases (mostly in patient whose immune system is otherwise compromised), can even develop in a full-blown infection.
Despite these concerns, this still constitutes the vaccine of choice for the protection against smallpox, presumably because efforts toward the development of an alternate method were stopped by the disease eradication in 1979. However concern about the use of smallpox as an agent of bioterrorism has lead to a renewal in the use of this vaccine for troops stationed overseas.
Use of inactivated agents and toxoid vaccines:
These constitute the bulk of vaccines. Essentially, you are taking your agent and killing it (either by formalin or heat treatment) and then injecting it into the body and letting the non-specific immune system pick it up and present it to the specific one. It is a common, comparatively easy to develop (grow your agent and then nuke it) and cheap method for the production of a vaccine. Jonas Salk’s success in developing a vaccine against polio relied on the use of a killed virus when previous, unsuccessful efforts had attempted to develop an attenuated strain of the virus.
It is not perfect; however, because the agent is inactivated, it will not colonize the mucosa. You have to inject it directly into the body, and not only can this be a problem for the most needle-phobic among us, but it also mean you are bypassing the whole mucosal compartment of the immune response (remember the various compartments do not really communicate with each other), so the cells in your first line of defense will be caught unaware. Moreover, because the pathogen is inactivated, it will not multiply within the body, which means, it won’t stimulate the immune system as well or for as long as an actual infection. For this reason, booster shots can be required.
A way around that problem, that helps to make sure that the immune system will notice the introduction of the antigen, is to inject it alongside an adjuvant — an immunogenic molecule that will help recruit cells of the innate immune system. The drawback of this method is that such molecules are going to cause inflammation at the site of injection, and can induce fever and other non-specific immune responses. Indeed, more often than not, the adverse effects that one experience from an inactivated vaccine are due to the adjuvant rather than the antigenic component of the vaccine.
Toxoid vaccines are pretty similar, except, in that particular case, rather than targeting the actual infectious agent, it is one of its toxins that is being denatured and injected. Tetanus and diphtheria toxins, for example, are the main toxins targeted by such vaccines.
Use of sub-unit, recombinant and conjugate vaccine:
As we mentioned a few times, the specific immune system is not presented with the whole pathogen, but only with its antigenic components.
Therefore, it is possible to only use the antigenic parts of the pathogen and still induce an immune response. These parts can be obtained either directly by purification lysates from the pathogen, or, more sneakily, in the case of recombinant vaccines, by looking in the pathogen genome for the genes that code for the antigens. Then, you can copy those genes and stick them on some innocent by-stander so that it will express the proteins in question. You then isolate the proteins from the culture, and inject that instead.
In some cases, the protein itself will not be recognized by the immune system, generally, because it is too small. In such a case, this protein, called a hapten, will be ligated to a larger molecule, called a carrier and it is the hapten-carrier complex that will be recognized by the immune system in order to create a conjugate vaccine.
The use of a sub-unit or conjugate vaccine is a bit more involved than that of a inactivated vaccine but it often allows the use of antigens from multiple strains of a pathogen. Also, it allows us to avoid certain element of the pathogen. For example, the caustative agent of whooping cough, Bordetella pertusis’s outer membrane harbors endotoxins that can cause adverse reactions by stimulating the immune system too much and producing an inflammatory response. Recently, a sub-unit preparation was developed that did not include the endotoxins, allowing for a safer vaccine that is now part of the DTaP (alongside diphtheria and tetanus). Because of the new availability of this safer alternative, the vaccination against pertussis is now recommended to adults and teenagers, especially considering their potential role as carriers and the recrudence of whooping cough numbers, that are reaching their highest levels of incidence in 50 years.
Use of live attenuated vaccines:
The last major type of vaccines is termed live-attenuated. There are a couple of method to obtain them. First, many virulence factors are only useful for the pathogen once it is in its host. After all, what use is the clot dissolving streptokinase when there are no platelets around? In fact, the metabolic expenses of expressing such virulence factor often become a drawback when the pathogen is cultivated in vitro. So, it is often observed, that after a few generations of being cultivated in vitro, many pathogen will lose some of their virulence, in a simple illustration of selection whose sight would warm Charles Darwin’s cockles (he actually did work on barnacles quite a bit).
The main area of concern in this context, is reversal to virulence. If the lack of selective pressure on the pathogen leads to the down-regulation of the virulence genes, one would expect that reestablishment of this pressure once the pathogen is introduced in the body might restore it. This is a logical concern, but also something that vaccine makers are aware of and keep an eye on, and the attenuated vaccines that make their way to the general public have been repassed so many time that they have accumulated a number of mutations in their virulence genes, making in unlikely (albeit not impossible) that the vaccine will revert to virulence.
An alternative method is to target the virulence genes directly and proceed to genetically engineer them away, for example, through genetic recombination. In this case, the risk of reversion to virulence is nil as the genes responsible are no longer present within the pathogen genomes.
In addition to this reversion to virulence, attenuated genes have a few drawbacks. They are more costly to produce. They are also more fragile than inactivated organisms making their distribution, especially within developing countries, more difficult and complicated, for example, they often require to be maintained under refrigerated temperatures. Furthermore, even when they remained attenuated, they can sometimes overcome the immune system of particularly immunodeficient patients. Nonetheless, they have a number of great advantages. Contrary to the inactivated vaccines, attenuated vaccines can colonize a mucosal epithelium, it is therefore possible to administer them, for example orally or nasally as is done for the attenuated version of the polio vaccine. Not only does it simplify administration, it allows the vaccine to stimulate the mucosal compartment of the immune system. They also tend to remain longer in the organism and are less likely to require a booster. Finally, in the case of the intra-cellular pathogen, they will retain their ability to invade host cells and stimulate the cellular component of the immune response.
DNA vaccines:
There is a last interesting vaccine method. This method is still experimental and, I suspect, will remain so for a long time. Essentially, it starts in a way pretty similar to a recombinant vaccine by making copy of the genes responsible for the expression of the antigens. Then, instead of expressing them in a third organism, these genes are conjugated to an expression vector (generally based on viral promoter such as a Herpesviridae or cytomegalovirus) and injected directly into the patient. Some of these DNA fragments will then be picked up by the patient’s own cells that will produce the proteins.
The advantages of this type of vaccines are numerous: there is no risk of infection, and it will stimulate both arms of the immune system. It lasts for a very long time and there is no need for adjuvant, and no molecules such as endotoxin, so the risks for inflammation are very low. These vaccines are also more stable than the attenuated ones and easier and cheaper to store and move around. There are also a few difficulties, but the main problem I suspect for its adoption will be people’s resistance to get themselves injected with viral DNA that will travel to their cells’ nucleii and get mixed with their own.
No words about autism?
So, I just spend the last seventeen hundred or so words talking about the various types of vaccines and their various drawbacks, and I have yet to acknowledge the elephant in the room: the often publicized relationship between vaccines and autism.
Why? Well, to put it bluntly, because there is every reason to think that no such link exists. The association between the two is pretty much entirely based on a faulty correlation: the first vaccinations occur at about the same time that the symptoms of autism become evident.
But, apart from this post hoc ergo propter hoc fallacy, there is little reason to believe the two are linked. Indeed, there are no theorical mechanisms through which vaccination would cause autism, as illustrated by the forever shifting rationales used by the vaccine opponents: from mercury toxicity (disproven and rendered moot when ethylmercury was removed from childhood vaccines) to Wakefield’s discredited “leaky gut” hypothesis (and at this stage, after Brian Deer’s latest revelations, I think that Wakefield himself qualifies as a discredited hypothesis), to the idea of an immune system overload. When investigated, these claims are systematically proven to be inaccurate and yet, the vaccines opponents simply migrate to another claim, showing that they already have decided that vaccines were the culprint and are now looking for a post-hoc rationalization.
In reality, there is good evidence that the often-touted autism epidemic is but an artifact of a subjective diagnostic and moving definition. After all, if the real incidence of autism was rising, one would expect that the rate of autism would be higher among younger children. But it is not the case, and the rates appear uniform among all age groups.
Yet, the connection persists in the collective mind. This is not difficult to see why. Raising a special child is taxing and difficult. It leads to anxiety and, too often, to an irrational feeling of guilt. What’s more, autism is a complex condition, without a clearly defined mechanism and cause, and this incertitude, this lack of answers, only adds to the distress of the parents.
In these circumstances, it is not surprising to see the appeal of the vaccine connection when it offers not only an answer but also a party to point the blame at and, on many occasions, the promise, however elusive, of a cure. There is something tragically human, eminently understandable, to this behavior, and one can do nothing but feel the deepest sympathy for the parents in this situation.
And yet… and yet it does not change the facts. On the contrary, because this issue is so charged emotionally, one has to cling to the scalpel-cold objectivity of science harder than ever. And the facts are that vaccines are, on the whole, very safe and very efficacious. That, while they do carry some risks of side effects (not autism, mind you, but other, real, risks), these risks are dwarfed a thousandfold by that of the actual diseases that vaccines protect against and that the world before the multiplication of vaccines, was a much grimmer place, full of cemeteries with tiny headstones — a world where too many diseases were endemic especially among younger children. In 1952, for example, the year Jonas Salk first tested his polio vaccine, 38,000 cases of infection by the virus were reported in the U.S alone, killing thousands and leaving more than 20,000 disabled. Today, vaccination has allowed this number to drop to about a thousand cases annually reported world wide, mainly in developing countries in Africa and Asia were the vaccination effort is impaired by war and extreme poverty.
Measles was similarly fought back: In the decade before the measles vaccination program began, an estimated 3–4 million cases were reported each year in the United States resulting in 400 to 500 fatalities and thousands of people left disabled from encephalitis. Nowadays, these numbers have been driven down to the mere hundreds of new cases annually and it is considered that the virus is no longer endemic in the U.S. And diphtheria which once claimed 13,000 to 15,000 lives annually (out of 100,000 to 200,000 cases) has only been reported about twice annually since the year 2000.
Yes, we must listen to the vaccine opponents and understand where they are coming from. But we also need to battle their misconceptions at every opportunity, because we are talking about lives, potentially thousands of them, being lost due to their well-meaning, misguided crusade, and that is simply a tragedy.
VII. Herd immunity and you! So, there are a few pathogens out there that can bury themselves deep into the body and remain there for a time. Mycobacterium tuberculosis, for example, can wall itself behind layers of layers of giant immune cells and remain there, in a dormant stage, for a time. Herpes simplex virus can enter a cell and express an RNA molecule, the LAT RNA, that down regulates not only the apoptotic mechanisms in the host cell but also several of the virus’ own genome, causing the virus to enter a dormant state and remain hidden for prolonged period of time. But, for most pathogens, there is no such dormant stage and the pathogen meets the immune system head on leading to one of two outcomes: either the immune system wins and the infection is cleared, or it loses, and the individual ultimately dies of the infection.
Clearly, most people would deem the first outcome preferable, but, from a purely epidemiological point of view, the two actually share a very important aspect: in both cases, the infected individual will stop carrying the infection and infecting others.
So the pathogen only has a limited window of time, once it infects a host, to jump into another one. This means that the number of people the host will meet and have the opportunity to transfer the pathogen too is going to be limited. Certainly, these numbers will vary quite widely depending on the particular pathogen in question, depending on how long the average infection takes, for example, and how easily it is transmitted. But, in all cases, the average number of new cases one infected patient will generate (the technical term is basic reproduction number) will be limited.
To take an easy example, let’s say that the pathogen’s “window of opportunity” (basic reproduction number) is five potential additional victims (see figure 6a). The infection is progressing very fast within the population, increasing the number of infected people by five every cycle.
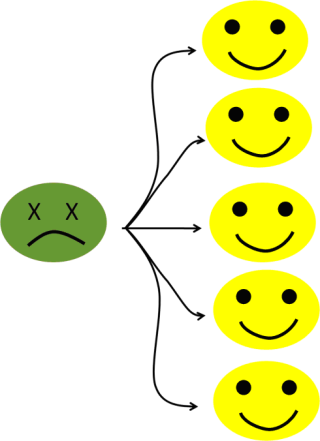
Figure 6a: Five potential secondary cases.
Now, let’s imagine that we have a way (wink, wink, I mean, using vaccines), to prevent the infection of, let’s say, 80% of the population (see figure 6b).
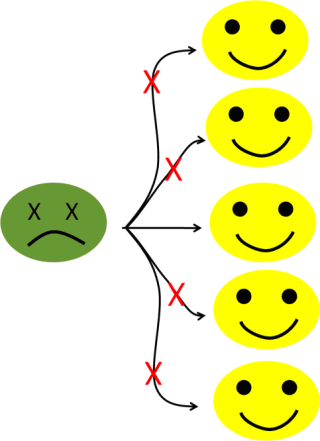
Figure 6b: With an 80% level of immunization, the number of potential secondary cases falls to one.
Now, we can see that the pathogen is only infecting one person. When the first (the index) case is cured (or dies, he doesn’t look so good, all green like that), then we will be back to the starting position, with just one infected patient.
Any higher level of protection and each infected patient will, on average, infect less than one new patient. Therefore, the number of infected persons will decrease with every cycle and the infection becomes self-limiting. As said, the size of the pathogen’s window of opportunity will vary depending on its virulence within the host or level of transmitability. So, the number of patients to vaccinate to reach the sweet point at which an infection is self-limiting will vary from pathogen to pathogen.
In general, the figure of 80% (that I choose mostly for simplicity’s sake) is actually not too far off the mark (see figure 7). It is in the low- to middle-eighties for mumps, rubella, polio and even measles. Pertussis, on the other hand, is a pretty highly contagious infection, with a basic reproduction rate between 12 and 17, requiring a high level of immunization (in the lower nineties) before herd immunity starts breaking down. This is, in fact, the only infection for which the levels of immunization within the population are significantly lower than the estimated required levels, and, unsurprisingly, it is the one we are seeing an epidemic of, right now (with, as I mentioned multiple times, the highest numbers of infection recorded in 50 years).
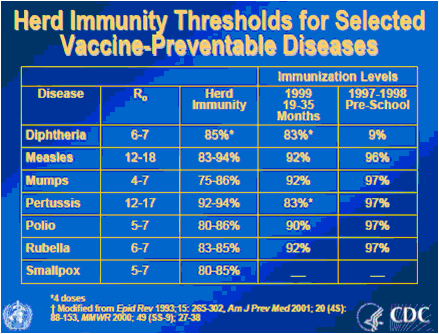
Figure 7: Basic reproductive rate and estimated levels of protection required to achieve herd immunity (from History and Epidemiology of Global Smallpox Eradication).
Now, vaccines do not always work. Sometime, one particular injection “does not take,” or some patients are unable to mount an immune response (AIDS patients) or the person was simply not vaccinated for some reason, maybe because he was too young. And sometime whole communities, for example, in the case of religious exemptions, are lacking vaccination.
But these persons are still going to be protected by vaccination, because, if enough of the population is protected, if herd immunity is achieved, the infection will wind down before reaching the non-protected individuals.
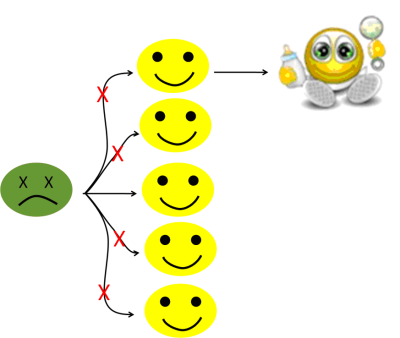
Figure 6c: Protected by herd immunity!
Now, if you will indulge me one comparison: it is well known that when Europeans arrived in America, smallpox was unknown on the continent. The natives were not immune, far from it, but they had no outbreak, because they had the whole ocean blue to isolate them from the Old World and its diseases.
In the case of vaccination, it is the ocean of vaccinated people that serve as a barrier between the pathogens and their potential victims, and every person that refuses a vaccine is inviting every microbial Christopher Columbus out there to use him as its Santa Maria.
And, that, my friends, is not good at all. We are currently starting to see, again, what happens when herd immunity breaks down, this is the lesson of today’s Pertussis epidemic, and that is a lesson that we need to take to heart fast, before any more kids die of an easily preventable death.
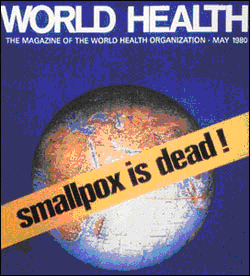
Figure 8: The eradication of smallpox, the greatest killer to ever afflict mankind stands as one of science’s greatest achievements.
Read or Add Comments about this Article
|
|
|
Back to Skeptillaneous
|